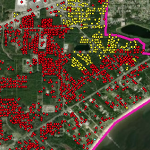
Multi-Hazard Hurricane Vulnerability Model to Enable Resilience-Informed Decision
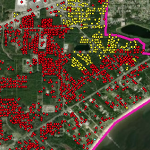
Then, to best utilize this new mesh, a multi-resolution approach is implemented to use meshes of varying resolution when and where it is required. It is hypothesized that by `switching’ from coarse- to fine-resolution meshes, with the resolution in the fine mesh concentrated only at specific coastal regions influenced by the storm at that point in time, both accuracy and computational gains can be achieved. As the storm approaches the coastline and the landfall location becomes more certain, the simulation will switch to a fine-resolution mesh that describes the coastal features in that region. Application of the approach during Hurricanes Matthew and Florence revealed the predictions to improve in both accuracy and efficiency, as compared to that from single simulations on coarse- and fine-resolution meshes, respectively.
Finally, the efficiency of the approach is further improved in the case of Hurricane Matthew, by using multiple smaller fine-resolution meshes instead of a single high-resolution mesh for the entire U.S. southeast coast. Simulations are performed utilizing predicted values of water levels, wind speeds, and wave heights, as triggers to switch from one mesh to another. Results indicate how to achieve an optimum balance between accuracy and efficiency, by using the above-mentioned triggers, and through a careful selection of the combination meshes to be used in the approach. This research has the potential to improve the storm surge forecasting process. These gains in efficiency are directly a savings in wall-clock time, which can translate into more time to invest in better models and/or more time for the stakeholders to consider the forecast guidance.
This research builds upon a process that uses maximum water elevation output from the Advanced Circulation (ADCIRC) model and downscales these results to a finer resolution by extrapolating the water levels to small-scale topography. This downscaling process is referred to as the static method. The method was originally designed for use in North Carolina (NC), where results from an ADCIRC model designed specifically for NC were downscaled to a set of NC topographical data. By joining the static method with an ADCIRC output visualization tool, the downscaling process is now able to run faster with the same level of accuracy and can run on any ADCIRC model with downscaling data from any geographical region or given resolution. This process is used to provide extra guidance to emergency managers and decision makers during hurricanes.
The downscaling process is also improved by adding physics using the slopes method and the head loss method. The slopes method incorporates the slopes of the water levels produced by ADCIRC, rather than only the value of the water level. By interpolating ADCIRC output water elevation points into a smooth surface, slopes of this surface can be used to influence the elevations of downscaled water levels. The head loss method adds friction loss due to variations in the ground surface based on land cover types and friction associated with each type. As water travels over any surface, head loss, or a loss in energy, occurs at different rates depending on the surface roughness. This rudimentary hydrologic principle is applied to increase the accuracy of the downscaling process at minimal cost. The downscaling methods are applied for results from an ADCIRC simulation used in real-time forecasting, and then compared with results from an ADCIRC simulation with 10 times more resolution in Carteret County, NC. The static method tends to over-estimate the flood extents, and the slopes method is similar. However, the head loss method generates a downscaled flooding extent that is a close match to the predictions from the higher-resolution, full-physics model.
By improving the accuracy of downscaling methods at minimal computational cost and expanding the applicability of these downscaling methods, these methods can be used by emergency managers to provide a better estimation of flooding extents while simulating storm events.