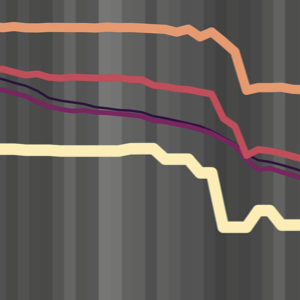
Resolution Sensitivities for Subgrid Modeling of Coastal Flooding
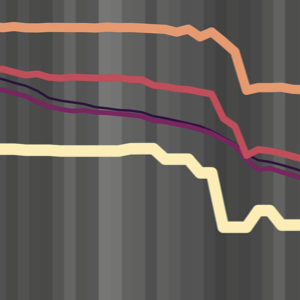
The water level (tide and surge) was simulated and the potential flooding resulting from historical hurricanes (Irene and Isabel) in Norfolk, VA was evaluated. The model was forced using the parametric Holland Model and various perturbations in the hurricane characteristics were evaluated. In addition, projected relative sea level rise up to the year 2150 was investigated.
D-Flow can accurately simulate the water level with an average correlation coefficient and root-mean-square-error of 0.974 and 0.17 m, respectively. Water level prediction showed high sensitivity to climate change impacts and inaccuracies in hurricane track and lower sensitivity to changes in hurricane central pressure and radius of maximum wind. A mesh resolution that reflects accurate topographical depiction is required to estimate the flood area accurately. Willoughby Spit (a narrow peninsula north of the naval base extending into Chesapeake Bay) was the most susceptible area to flooding. Significant parts of the base were found to be vulnerable to flooding under the considered scenarios, with flood areas ranging from 0.28 km2 to 5.94 km2 (1.3%–43% of the base area), with the largest predicted flooding for the sea level rise and wind speed scenarios. The insights of the sensitivity of flood predictions to various factors could enable targeted adaptation measures and resource allocation, for enhanced resilience and sustainable development in vulnerable coastal areas.
In this research, we implemented a neural network to predict peak values for total water level (tides and storm surge) at multiple stations, considering astronomical tides and storm tracks of any duration as inputs. To create the training library, we simulated 1,813 synthetic tropical cyclones based on historical data in the North Atlantic Ocean, with a specific focus on storms that affect North Carolina. These simulations used a full-physics hydrodynamic model with variable spatial resolution of about 50 m near the coast. The outputs were downscaled to grayscale images with a higher and constant resolution of 15 m, enhancing the flood predictions by considering small-scale topographic features, and then used as training data for the neural network. The many-to-one deep learning model predicts a single peak total water level in time at multiple locations in space using time series of the offshore astronomical tide and track parameters as inputs. We used the model to make probabilistic predictions of peak total water levels for observed and perturbed tracks of several historical storms that affected North Carolina.
We showed that the neural network performed well (with errors ranging from 8 to 43 cm) in predicting peak total water levels at nine locations in North Carolina. We applied the neural network to make probabilistic predictions of peak total water levels for observed and perturbed tracks of historical storms. For each storm, the neural network predicted at nine stations for 101 storm scenarios (the true/historical storm and 100 perturbations) in less than 10 seconds. The performance for the observed historical storms was similar to those obtained in process-based simulations, but with a significant gain in computational runtime.
In this dissertation, subgrid corrections have been added to the ADvanced CIRCulation (ADCIRC) model, a widely used, continuous-Galerkin finite-element based, shallow water flow model. This includes the full derivation of averaged governing equations, closure approximations, and subgrid implementation into the source code. Testing of this new model was first performed on 3 domains: an idealized winding channel, a tidally influenced bay in Massachusetts, and a regional storm surge model covering Calcasieu Lake in Southwestern Louisiana with forcing from Rita (2005). By pre-computing the averaged variables from high-resolution bathy/topo data sets, the model can represent hydraulic connectivity at smaller scales. This allows for a coarsening of the model and thus faster predictions of flooding, while also improving accuracy. The implementation permits changing a logic-based wetting and drying algorithm to a more desirable logic-less algorithm, and requires averaging correction factors on both an elemental and vertex basis. This new framework further increases efficiency of the model, and is general enough to be used in other Galerkin-based, finite-element, hydrodynamic models. It is shown that the flooding model with subgrid corrections can match the accuracy of the conventional model, while offering a 10 to 50 times increase in speed.
Next, higher level corrections to bottom friction and advection were incorporated into the subgrid model, and the framework was expanded and tested at the ocean-scale. It was hypothesized that by adding higher-level corrections to the model and applying them to ocean-scale domains, accurate predictions of storm surge at the smallest coastal scales can be obtained. To accomplish this, higher-level corrections were derived and implemented into the governing equations and extensive elevation and landcover data sets were curated to cover the South Atlantic Bight region of the U.S. Atlantic Coast. From there, the subgrid model was tested on an ocean-scale domain with tidal and meteorological forcing from Matthew (2016). The improvements in water level prediction accuracy due to subgrid corrections are evaluated at 218 observation locations throughout 1500 km of coast along the South Atlantic Bight. The accuracy of the subgrid model with relatively coarse spatial resolution (RMSE = 0.41 m) is better than that of a conventional model with relatively fine spatial resolution (RMSE = 0.67 m).By running on the coarsened subgrid model, we improved the accuracy over efficiency curve for the model, and as a result the computational expense of the simulation was decreased by a factor of 13.
Finally, subgrid corrections were systematically tested on a series of five ocean-scale meshes with minimum nearshore resolutions ranging from around 60 m on the highest resolution mesh to 1000 m on the coarsest mesh. This study aimed to find the mesh resolution that offered the best trade-off between accuracy and efficiency. The limitations of the subgrid model were explored and guidelines for future users were established. In all, it was found that the primary limitation to the subgrid model came from the aliasing of important flow-blocking features such as barrier islands in the coarsest resolution meshes. However, in areas without these features subgrid corrections can offer tremendous advantages while running on very coarse meshes.
The work completed in this dissertation moves the science of subgrid corrections forward by integrating the corrections into a widely used ocean-circulation and storm surge model. This work offers improvements to both hurricane storm surge forecasting and long-term design by allowing for reduced run-times and increased accuracy on coarsened numerical meshes.