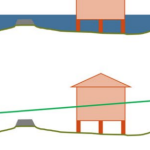
Downscaling of Real-Time Coastal Flooding Predictions for Decision Support
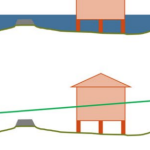
Then, to best utilize this new mesh, a multi-resolution approach is implemented to use meshes of varying resolution when and where it is required. It is hypothesized that by `switching’ from coarse- to fine-resolution meshes, with the resolution in the fine mesh concentrated only at specific coastal regions influenced by the storm at that point in time, both accuracy and computational gains can be achieved. As the storm approaches the coastline and the landfall location becomes more certain, the simulation will switch to a fine-resolution mesh that describes the coastal features in that region. Application of the approach during Hurricanes Matthew and Florence revealed the predictions to improve in both accuracy and efficiency, as compared to that from single simulations on coarse- and fine-resolution meshes, respectively.
Finally, the efficiency of the approach is further improved in the case of Hurricane Matthew, by using multiple smaller fine-resolution meshes instead of a single high-resolution mesh for the entire U.S. southeast coast. Simulations are performed utilizing predicted values of water levels, wind speeds, and wave heights, as triggers to switch from one mesh to another. Results indicate how to achieve an optimum balance between accuracy and efficiency, by using the above-mentioned triggers, and through a careful selection of the combination meshes to be used in the approach. This research has the potential to improve the storm surge forecasting process. These gains in efficiency are directly a savings in wall-clock time, which can translate into more time to invest in better models and/or more time for the stakeholders to consider the forecast guidance.
NSWEEP=1
.
In late May 2019, the SWAN developers released a new version. Whenever this happens, the new version needs to be implemented into the coupled SWAN+ADCIRC, thus replacing an older version in the coupled model.
Starting with the upcoming release version 55 of ADCIRC, the coupled SWAN has been upgraded to its latest release version 41.31. It replaces the older version 41.10.
This upgrade is mostly a benefit to users of SWAN+ADCIRC. It has been almost 4 years since the last upgrade, and we had skipped a new SWAN version (41.20) during that time. Thus, this upgrade is adding features and bug fixes from two newer versions (41.20 and 41.31). SWAN has added several capabilities that will be advantageous to users of SWAN+ADCIRC.
However, a few of its changes will cause differences in the wave predictions, as described below. Users will likely need to re-calibrate their input settings for SWAN.
Originally developed as a tool for visualizing ADCIRC output, Kalpana has evolved to include methods for downscaling ADCIRC water elevation results. The first method, now referred to as the static method, extrapolated ADCIRC water elevations horizontally until intersecting an equivalent DEM elevation. More information about the static method and about downscaling ADCIRC results with Kalpana can be found on an earlier post.
The static method has proven to be a useful tool but incorporates minimal physics. Therefore, a new method, referred to as the head loss method, has been introduced to include energy dissipation due to land cover during overland flow events. In this page, we describe the theory of the head loss method and provide examples for how to apply it using Kalpana.
Side-view schematic of downscaling methods. A one-dimensional schematic is displayed for each of the two downscaling methods, where the top figure is the static method and the bottom is the head loss method. In the static method, the water elevations from ADCIRC (blue hatched portion) are extrapolated as a flat surface until they intersect the DEM. In the head loss method, these water elevations are extrapolated to an energy cost surface (elevation plus cumulative head loss).