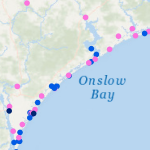
To predict storm surge and its associated flooding in coastal areas, numerical models must have a detailed representation of the impacted region, and thus be accurate, without having too much detail, which can limit efficiency. This research examines the use of a multi-resolution approach to improve both efficiency and accuracy. The key idea is that, as a storm travels across the ocean, it will affect different regions at different times. Early on, as the storm moves in open water and far from people, efficiency is more important in the model predictions. But as the storm moves toward the coast, it becomes necessary to have a higher accuracy near coastal communities and infrastructure. This research examines the use of multi-resolution simulations in which, as a storm travels along its track, the model ‘switches’ from lower resolution in open water to higher resolution as the storm moves closer to land. The main research question is to determine when is it most beneficial to switch resolutions by determining when storm effects are first seen at the coast.
This research will explore the arrival of storm effects for Florence, which made landfall along the North Carolina coast during September 2018. It is an ideal storm for this research as its track was shore-normal, and thus its coastal effects increased as it approached landfall. This will allow for investigating the most optimal switch by focusing on a single switch between a lower-resolution mesh to a higher-resolution mesh. The switches will be initiated by several triggers, including wind speeds and water levels at the coast and inland locations, and with several lead times, including near and several days before landfall. Model performance will be quantified via comparisons to observations of storm effects in the region, as well as to a single, high-resolution simulation for the full storm. It will be shown that switching from a coarse resolution mesh to a fine resolution mesh will lead to an increase in efficiency gains across all switching simulations with the most optimal switch time resulting in the most accurate predictions of water levels as compared to our full high-resolution simulation.
The results of this research will provide valuable contributions to forecasters working tirelessly during hurricane season to produce accurate and efficient predictions of coastal flooding impacts. With this information, real-time forecasts can be delivered sooner to emergency managers for informing evacuation zones, thus saving lives.